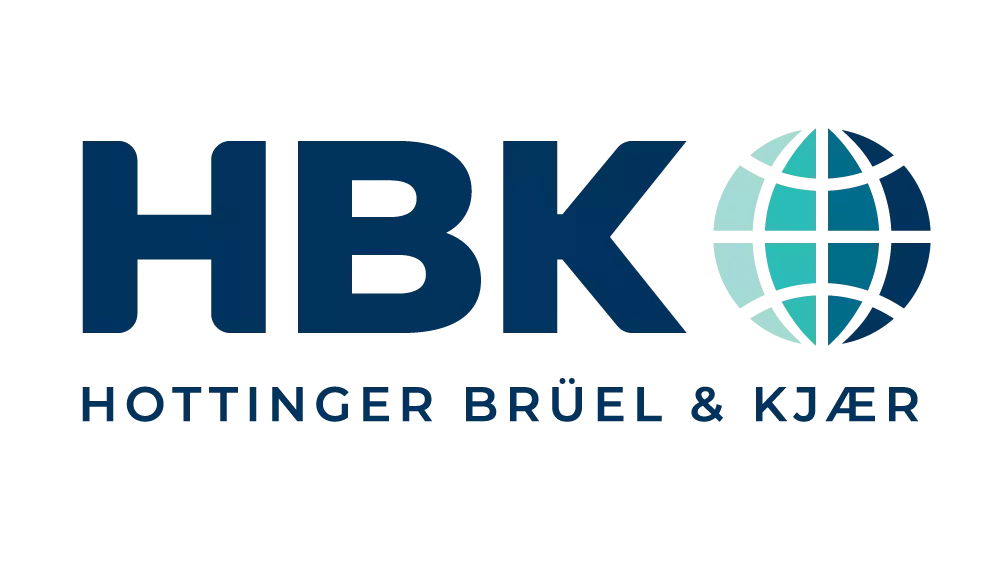
Before the right strain gauge can be selected, the measurement goal needs to be clearly set. The main question to be considered is whether the strain gauge will be used for experimental tests, durability tests or for transducer manufacturing.
|
|
Strain gauges for experimental tests | Strain gauges for transducer manufacturing |
The strain gauge selection for experimental test will be done regarding the following selection criteria:
1. Geometry: | Number and position of grids (pattern) |
2. Strain gauge series: | Construction of strain gauge |
3. Connections: | Type and position |
4. Temperature response adaptation: | Material to which strain gauge temperature response is matched |
5. Active grid length: | in mm |
6. Electrical resistance: | in Ohm |
![]() | Linear strain gauges (e.g. LY4) have one measuring grid and measure the strain in one direction. |
![]() | T rosettes (e.g. XY3) have 2 measuring grids arranged at a 90° degree offset from each other. Typical applications for this strain gauge type include analysis of a biaxial stress state with known principal directions as well as measurements on tension and compression bars. |
![]() | V-shaped strain gauges (e.g. XY4) have 2 measuring grids arranged at a 90° degree offset from each other. Typical applications for these strain gauges include measurements on torsion bars and determining shear stresses as they occur in shear beams in the area of neutral fibers. |
![]() | Double linear strain gauges (e.g. DY4) have two measuring grids arranged parallel to each other. Typical applications for these strain gauges include measurements on bending beams. |
![]() | Rosettes with 3 measuring grids (e.g. RY8) arranged at an angle of 0°/45°/90° or 0°/60°/120° are an appropriate choice for analyzing the biaxial stress state with unknown principal directions. |
![]() | Strain gauge chains (e.g. KY8) consist of 10 or 15 very small measuring grids that are placed on a common carrier at a constant spacing (equidistant apart) plus one compensation SG. Strain gauge chains are especially suitable for determining strain gradients. |
![]() | Full bridge strain gauges (e.g. VY4) have 4 measuring grids, which are arranged so that each is offset to the next at an angle of 90°. Typical applications for full bridge strain gauges include measurements on tension/compression bars and determining shear stresses as they occur in shear beams. |
For experimental tests robust and flexible strain gauges, which can be used under arduous conditions, have distinct advantages. Strain gauges having the synthetic, polyimide, as carrier material for the measuring grid and with the series identification "Y" are in this category. This series contains a large number of different types of strain gauges used for a variety of tasks in experimental tests. There are also numerous special types of strain gauges, e.g. hole-drilling and ring core rosettes for the determination of residual stresses in structural parts and strain gauge chains for the investigation of stress distribution on complex structures.
![]() |
Integrated solder tabs (e.g. LY4)
|
![]() |
Big solder tabs with strain relief (e.g. LY6)
|
![]() |
Ni-plated copper leads, uninsulated, approx. 30 mm (1.18 inch) long (e.g. LY1)
|
![]() |
Fluoropolymer-insulated connection wires, approx. 50 mm (1.97 inch) long (e.g. K-C LY4)
|
![]() |
Fluoropolymer-insulated connection wires, approx. 50 mm (1.97 inch) long (e.g. K-C LY4)
|
Strain gauges that are connected individually in a Wheatstone quarter bridge circuit will show an output signal if the temperature changes. This signal is called “apparent strain” or “thermal output” and is independent of the mechanical load on the test object.
However, it is possible to adjust a strain gauge to the thermal expansion coefficient of a specific material, such that the output signal is very small in case of a temperature change. Such strain gauges are called strain gauges with “matched temperature response” or “self-compensated” strain gauges.
To benefit from their matched temperature response, strain gauges must be selected to the thermal expansion coefficient a of the test material.
Code | Material | Thermal expansion coefficient ∝ |
1 | Ferritic steel | 10.8 ⋅ 10-6/K (6 ⋅ 10-6/°F) |
3 | Aluminum | 23 ⋅ 10-6/K (12.8 ⋅ 10-6/°F) |
5 | Austenitic steel | 16 ⋅ 10-6/K (8.9 ⋅ 10-6/°F) |
6 | Quartz glass / composite | 0.5 ⋅ 10-6/K (0.3 ⋅ 10-6/°F) |
7 | Titanium / gray cast iron | 9 ⋅ 10-6/K (5 ⋅ 10-6/°F) |
8 | Plastic | 65 ⋅ 10-6/K (36.1 ⋅ 10-6/°F) |
9 | Molybdenum | 5.4 ⋅ 10-6/K (3 ⋅ 10-6/°F) |
Long measuring grids are recommended where there is an inhomogeneous material, such as concrete or wood. A long strain gauge will bridge the inhomogeneities of the work piece and, as a measurement result, will supply the strain underneath the measuring grid.
Short measuring grids are suitable for detecting a state of local strain. They are therefore suitable for determining strain gradients, the maximum point of notch stresses and similar stresses.
Low Ohm strain gauges | High Ohm strain gauges |
+ Lower influence of electromagnetic interferences | + Lower influence of electrical resistance in connection paths (slip-rings, cables,...) |
+ Lower influence of a change in isolation resistance | - "Better" antennas in the case of interferences |
- Higher power requirement | - Higher influence of a change in isolation resistance |
- More self-heating due to higher current flow compared to high Ohm strain gauges |
Influence of resistance and other parameters on the temperature at the measurement point
This will bring together HBM, Brüel & Kjær, nCode, ReliaSoft, and Discom brands, helping you innovate faster for a cleaner, healthier, and more productive world.
This will bring together HBM, Brüel & Kjær, nCode, ReliaSoft, and Discom brands, helping you innovate faster for a cleaner, healthier, and more productive world.
This will bring together HBM, Brüel & Kjær, nCode, ReliaSoft, and Discom brands, helping you innovate faster for a cleaner, healthier, and more productive world.
This will bring together HBM, Brüel & Kjær, nCode, ReliaSoft, and Discom brands, helping you innovate faster for a cleaner, healthier, and more productive world.
This will bring together HBM, Brüel & Kjær, nCode, ReliaSoft, and Discom brands, helping you innovate faster for a cleaner, healthier, and more productive world.